ABSTRACT Spaceflight research about living systems in weightless environments describes gravity as an instructive force directing the growth and development of biological systems. Dr. Ida Rolf was saying this back when spaceflight was in its infancy in the 1970s. Current research describes the consequences of microgravity on the human body and its tissues, making space exploration research an area that Rolfers and structural integration (SI) practitioners might want to read more of. This summary of two peer-reviewed articles describes the absence of gravity on the human body, so that we may deepen our understanding of the presence of gravity and the human body.
In the profession of structural integration (SI), we have the expertise of being able to see with our eyes, feel with our body, and influence with our touch and verbal suggestions, a person’s organizational relationship with gravity. This is the teaching of Ida P. Rolf, PhD (1896-1979), she put gravity at the center of her fascial manipulation approach (1986), and SI practitioners trained in her tradition share this fundamental question – How is the client’s body organizing in Earth’s gravitational field?
Thanks to the interest in regular spaceflights, there is an abundance of microgravity research investigating what happens to the human body when it lives in a weightless environment, for short and long periods of time. SI practitioners have much to gain by reading and thinking about these findings. Astronauts experience a functional decline in their muscle strength and bone density during a six-month stay on the International Space Station (ISS). What a weightless environment is taking away can become instructive as to what gravity is giving to all of us on the Earth’s surface.
Every day of our lives, we relate with gravity. This is what Rolf taught. Gravity is an organizing force for human beings. It can be felt as balancing on our feet with ease and comfort. And the opposite can be true, that a person’s body can be disorganizing within their own weight, the measure of gravity collapsing and compromising tissue that needs a particular amount of space to function. This July ‘Fascia Insights’ column is an exploration of two academic articles about the effect of microgravity (the weightlessness experience during spaceflight) on humans, animals, and cultured mammalian cells. Rolf deduced and taught human organization patterns in gravity in the 1970s, and now we can learn from biological spaceflight studies an even more detailed picture of gravity’s effect on the human body.

People are able to live for days and months in the absence of gravity, as long as they have the necessities of life (breathable air, food, etc.). Yet, the longer an astronaut is in space, six months or longer1, the more they will have significant deficits emerge when they return to Earth. When astronauts return from space, they experience cardiovascular deconditioning (a decrease in heart muscle strength, a decrease in blood vessel flow rates, and low blood pressure), bone demineralization, muscle atrophy, and a continuum of other physiological changes. Add to that, animal and tissue studies indicate that “space is still not a place for either regular visits or habitation largely due to the challenges posed to normal growth and development of organisms” (Hariom et al. 2021, 522).
The absence of gravity isn’t immediately fatal. The effect of microgravity experienced during space missions by the human body is slow. It is a transient decline of structure and function, and there is significant suffering endured when people return to regular gravity. Upon returning to Earth, astronauts cannot stand up due to orthostatic intolerance. That is to say, when they stand, their cardiovascular system is unable to pump blood uphill to the heart and brain, so they feint. Even when astronauts work out while in space, frequent aerobic and resistive exercise is insufficient to prevent the deconditioning of various body systems. That is why astronauts returning from months on the ISS have special chairs to sit in while being interviewed right after landing back on Earth, their bodies need to heal from the stressors of space and the adaptations their systems have made.
The following summaries of microgravity research articles are an invitation to be curious about what spaceflight research can offer the SI community.
Hariom et al. 2021 – Animal Physiology Across the Gravity Continuum
Hariom et al. (2021) report that there has been an exponential increase in the number of studies on the effects of gravity on living systems. Mostly funded by the increased interest in long-term spaceflight, laboratories around the world are looking at biological systems in the microgravity environment, like the work being done on the ISS. But also, scientists are placing rats in special cages with food and water, in centrifuges for prolonged periods of time. Spinning the rats in the centrifuges simulates an increase of gravity to twice Earth’s gravitation force. Hariom et al. (2021) is a review article of both space and Earth laboratory studies, they looked at the effect of space’s microgravity and the centrifuge’s hypergravity on animal physiology. These authors conclude that gravity is instructive to biological systems. Gravity directs the orientation of growth and development within our cellular systems.
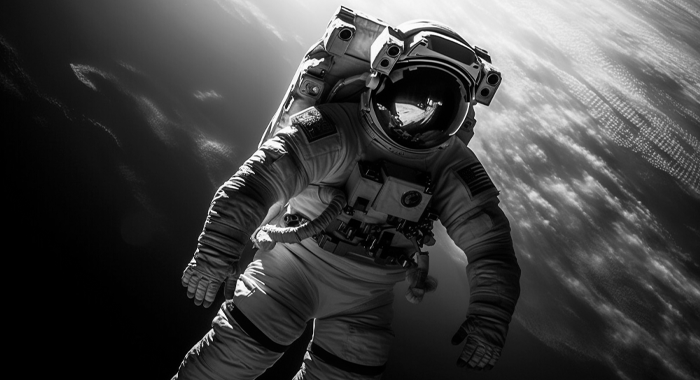
Cardiovascular System
Following the structure of their article, Hariom et al. (2021) summarized what is known about the cardiovascular deconditioning syndrome that astronauts experience. When we are standing here on Earth, and when we are watching our clients stand at the beginning of our SI sessions, part of what we are feeling and seeing is blood naturally pooling in the legs. Gravity pulls all the liquids of the body to the inferior aspect of their fascial containers, and for the cardiovascular system, blood pools in the legs. The human leg and heart are designed for this continuous downward pull. The heart muscle robustly pumps blood so that the pressure pushes the blood in the legs back up to the heart. The veins of the legs get the deoxygenated blood back to the heart with their series of valves preventing backflow.
Because the blood is being pulled downward, there is only so much blood that can be in the heart at one time. That downward pull on blood in particular maintains our arterial blood pressure. This gravity-induced pooling of the blood in the feet is described as a hydrostatic gradient, with high pressure in the circulatory system in the lower body while relatively lower pressure within the brain and heart. The cardiovascular regulatory mechanisms that monitor for the successful circulation of blood expect these conditions.
While in spaceflight, astronaut bodies lose their hydrostatic gradient. In the absence of the blood being pulled to the feet and legs, there is a fluid shift in the whole body from the lower body to the upper body. Astronauts will have puffy faces and skinnier than usual legs. The cephalad fluid shift can create numerous difficulties that increase over time.
Within the blood vessels themselves, endothelial cells compose the deepest layer of cells that line the inside of the tube. Endothelial cells are responsible for the flow of nutrients out of the vessels and into the extracellular matrix, as well as the flow of cellular byproducts out of the extracellular matrix and into the blood for excretion by the organs. In microgravity, blood vessel endothelial cells undergo morphological and physiological changes. These changes point to something fundamentally different with the cytoskeleton of these cells that they can’t hold the same shape, nor maintain the same physiological activity.
The Immune System
People who experience spaceflight are found to be infection-prone while in space and when they return home. Their systems have a remarkably reduced state of resistance to common infections. The immunosuppression seems to correlate with a reduction in the number of various immune cells. The cells of the immune system are responsible for recognizing foreign bacteria, viruses, and injuries, then responding by destroying the infectious agent and/or repairing the site of injury. To do this, immune cells move around, often like amoebas, reaching and squishing through the fascial matrix. In microgravity conditions, immune cells have compromised cytoskeletal integrity, they have less actin within their cells (one of the building blocks of the cytoskeleton), and it is thought this significantly affects the ability of these cells to migrate through the tissue and respond to infections.
Bone
Focusing on bone tissue, astronauts have pronounced and systemic bone loss after spending long periods of time in space. Weight-bearing bones have the most accelerated bone loss when compared to non-weight-bearing bones. On Earth, our legs are subjected to dynamic mechanical loading as we go about the activities of our lives. Within our bones, we have cells called osteoblasts that respond to the loading by making more hard bone. While we also have osteoclasts, which are cells absorbing bone tissue to make calcium available for the whole body.
In microgravity, there is a significant decrease in the mechanical loading experienced by the legs and trunk of the body. As already mentioned, even with exercise in space, bone loss will be significant after spaceflight. It is thought that the osteoblasts are disrupted by the absence of gravity and that there is a failure to make new bone at the same rate as when on Earth. Osteoblast cells detect gravity as a mechanical signal that leads to the biochemical response of building hard bone. Without gravity triggering those sensors, the osteoblasts are not getting the signal to maintain dense, hard bones. Osteoclasts also have reduced activity in microgravity, which is a problem because the calcium and other molecules that osteoclasts make available in the bloodstream are essential for bodily functions elsewhere.
The calcaneus, navicular, and talus had extensive bone erosion at the sites of leg muscle insertions. It was clear that site-specific bone remodeling was taking place where there was an absence of postural tension. Right where the body was expecting the muscular attachments to the weight-bearing bones to have tension, bone erosion was significant. In the absence of postural tensions, foot bones were weakened.
Muscles
Microgravity conditions are associated with a loss of muscle mass, a decrease in strength, and poorer functional performance. Researchers report that when astronauts go to space, the musculoskeletal system experiences mechanical unloading that results in a decrease in muscle protein synthesis, muscle atrophy, and an accelerated breakdown of muscle proteins. Mice that were in microgravity for fourteen days had an 8% to 9% decrease in muscle mass, and the myofiber cross-section of extensor digitorum longus and gastrocnemius had a 10% to 35% decrease in muscle mass.
Researchers have made special note that it is the antigravity postural muscles that have the greatest decline in muscle mass. Specifically, the soleus, adductor longus, and vastus intermedius were observed to be significantly smaller muscles after spaceflight. The mechanical unloading state of microgravity led to a decrease in protein synthesis within these tissues and cells, but also an increase in protein degradation. Gravitational unloading of postural muscles also altered the pattern of neuromuscular activation. While unloading of non-postural muscles had negligible effects on the pattern of neuromuscular activation.
Interestingly, the diaphragm was unaffected by the unloading of weightlessness. Due to the functional role associated with breath, these muscle fibers did not atrophy over time. Also, rats placed in twice the amount of normal gravity had twice as much muscle mass.
The Vestibular System
Of course, the vestibular system is altered in the microgravity of space, with the most prominent symptom experienced by astronauts being space motion sickness (SMS). The symptoms include nausea, dizziness, vomiting, sweats, feeling cold, and general paleness of the skin.
The vestibular system consists of twin structures, one located within each temporal bone. It is part of the left and right inner ear, and detects the acceleration of the head. There are two components – the semicircular canals and the otoliths. The former is a sensory organ that conveys the rotational movements of the head, while the latter relates linear accelerations. The otolith is a gravity-sensing organ because it monitors the linear direction of up and down in relation to the head being upright. The other linear direction the otolith monitors is the horizontal movement of the head. It is thought that when an astronaut turns their head while in space, their semicircular canals detect the rotation easily. Yet because the otolith is not registering the downward signal of gravity, the signal from the otolith to the brain is altered. The sensory mismatch between these two inputs may be why people experience vestibular disorientation.
Over time, astronauts report that SMS symptoms resolve the longer they stay in orbit. It has been measured that the number of synaptic contacts within the vestibular system neurology increase. This is likely an adaptation where the brain engages its plastic nature, a synaptic reorganization occurs where there is learning about the new messages from the otolith, and the brain integrates the new information environment. The otolith adaptation is likely why the SMS symptoms ease over time for long spaceflights (Hariom et al. 2021).
The Nervous System
People’s nervous systems do function in space. As demonstrated by Commander Chris Hadfield, who spent 144 days in space in charge of the ISS and performed David Bowie’s Space Oddity while there.2 Yet some big changes occur. There is an upward shift in the location of the brain within the calvarium, the cortex structures narrow, and the cerebrospinal fluid within the arachnoid space right at the very top of the skull is also narrowed in microgravity. These structural changes increase with the length of time in space.
As already mentioned, spaceflight induces a cephalad shift of body fluids, which increases intracranial pressure. Weightlessness disrupts the natural outflow of cerebrospinal fluid and venous blood from the skull. This is not great. The brain responds with increased sympathetic activity and increased synaptic plasticity. Energy consumption accelerates in the central nervous system to counter these microgravity stressors. As the pressure in the head increases, various visual issues can develop due to the pressure on the optic nerve, among other factors. All of this leads to higher oxygen consumption in the brain, which increases oxidative injury from the free energy released from all this activity.
Also, within the brain and throughout the nervous system, the blood vessels are lined with vascular smooth muscle cells that influence minute vasodilation and contraction associated with thinking, coordination, and perception, among other processes. Microgravity is known to lead to reduced muscular function of these blood vessels, and with altered regulation of cerebral blood flow, people experience impaired thinking, learning, and memory. This phenomenon increases over time. Living in a weightless environment alters neurotransmitter release that can have an associated behavior change. Microgravity also disrupts the blood brain barrier, that special lining of cells that protects the brain from infections from other parts of the body, making the brain more vulnerable to microbes and viruses.

Summary of Hariom et al. (2021)
It is stressful for living systems to be in space. Experiencing the environmental change from Earth’s gravitational force to being in the weightless environment of spaceflight induces an adaptive response to the physiology of the body. In microgravity, a person experiences compromised blood flow, both from less strength in the heart muscle and also changes in the blood vessels. In gravity, we have weight which induces tension and resistance in the body. These mechanical forces involved in standing and walking support the body to build a robust heart and strong smooth muscles in the blood vessels.
Thinking about this from a SI perspective, if microgravity induces a sympathetic nervous system stress state, then gravity gives us an opportunity to have rest with increased parasympathetic function. Our hearts are soothed and strengthened by the hold of gravity. Our blood is expected to be in our lower body, the human system knows how to manage blood pressure in the presence of this downward pull on our fluids. Our bones need this downward pull, osteoblasts rely on detecting dynamic forces to build stable weight-bearing bones. Muscle fibers need the constant mechanical load of gravity to organize their physiological functioning. And all these systems within gravity’s field, contribute to the balance of fluids for optimal functioning.

Wu et al. 2021 – Cells Respond to Space Microgravity Through Cytoskeleton Reorganization
As already mentioned, the human body has a gravity-sensing organ – the otolith. Thanks to microgravity research, now we know that each and every cell in our body is also detecting gravity. Wu et al. (2021) discuss the convergence of results from over forty spaceflight cellular biology studies. Changes to the cellular cytoskeleton appear to be a key factor involved in the myriad of cellular problems observed. They propose that here on Earth, our cells have a cellular mechanical equilibrium established by the various fibers of the cytoskeleton, and they require gravity to function optimally. When cytoskeleton equilibrium is broken by the gravitational change from ground to space, what follows are cellular morphological changes that fundamentally change muscle cells, cardiac cells, and immune system cells.
Before describing Wu et al. (2021), allow me to set the cellular stage, to give context to these cytoskeleton findings. From the perspective of any cell in your body, molecular equilibrium is death. If there were an equal distribution of any of the molecules of life (oxygen, glucose, sodium, chloride, calcium, as examples), then life for that cell would have ended. Life depends on gradients of concentration, there has to be separations between high concentrations and low concentrations of the biomolecules. The cellular membrane is a semi-permeable membrane that is a defining structure that separates cellular spaces from extracellular spaces. Fascia is another space-creating structure that contributes to gradient differentials within the body. Inside a cell, the cytoskeleton creates micro-spaces within the cytosolic fluid.
Cells stockpile some molecules all the time; cells rely on being able to create places of high concentration inside their cytosol. For example, muscles need many amino acids to make the protein structures that build muscle tissue. These cells actively pull in amino acids from the bloodstream, like a factory stockpiling material for its production line. Just like a production line, the cell produces loose columnar arrangements of organized fluid while making its proteins.
There are many production lines within the cell, side by side. These molecules are ‘floating’ around in the cytoplasm, free to move in any direction. According to these microgravity studies, cytoskeleton structures create spaces in relationship with gravity where the weight of the molecule, combined with the cytoskeleton spaces, produces columns of protein production that are organized within the cell. Gravity pulls on the individual masses of each of the production line molecules and the cytoskeleton fibers. The force of gravity and the weights of these molecules are likely directing where these tiny cytoskeleton fibers get physically placed. In the absence of gravity, the cell can still do its job, but the cytoskeleton gets shifted. The organized columns, the production lines of cellular materials, experience fluid shearing forces that disrupt their physical location in the cell as the human bodies travel from gravity to microgravity (Wu et al. 2021). It is not fatal to the person. Over time, as you will see, there are cellular functional changes that become more pronounced.
One last detail when imagining a cellular environment, all these molecular events are happening very quickly: the building of the cytoskeleton, the reconstruction of the cytoskeleton for cells that move around, and the building of proteins. The rhythm of the cell is fast-paced; it is a very rapid series of molecular events that have lots of kinetic, potential, and thermal energy. Free energy is the term biochemists use to describe these various types of energy within the body that we commonly think of as heat and the frenzy of molecular movement. My favorite biochemistry textbook captures this best:
Life Requires Free Energy
A living cell is a dynamic chemical system, operating far from chemical equilibrium. For a cell to grow or to make a new cell in its own image, it must take in free energy from the environment, as well as raw materials, to drive the necessary synthetic reactions. This consumption of free energy is fundamental to life. When it stops, a cell decays toward chemical equilibrium and soon dies.
Picture the molecules in a cell as a swarm of objects endowed with thermal energy, moving around violently at random, buffeted by collisions with one another. To specify genetic information – in the form of a DNA sequence, for example – molecules from this wild crowd must be captured, arranged in a specific order defined by some preexisting template, and linked together in a fixed relationship.
The bonds that hold the molecules in their proper places on the template and join them together must be strong enough to resist the disordering effect of thermal motion. The process is driven forward by the consumption of free energy, which is needed to ensure that the correct bonds are made, and made robustly. In the simplest case, the molecules can be compared with spring-loaded traps, ready to snap into a more stable, lower-energy attached state when they meet their proper partners; as they snap together into the bonded arrangement, their available stored energy – their free energy – like the energy of the spring in the trap, is released and dissipated as heat. In a cell, the chemical processes underlying information transfer are more complex, but the same basic principle applies: free energy has to be spent on the creation of order (Alberts et al. 2008, 8).

The Details of Wu et al. (2021)
Space cellular biology laboratories from all over the world have been sending numerous kinds of cells into space, to be studied both in space and back on Earth when they return. What researchers have observed is that space microgravity changes the shape of cells, how well they reproduce, alters their ability to differentiate, their cell-cell adhesion was compromised, they couldn’t move as well as they did on Earth, and communication pathways were disrupted. Wu et al. (2021) report that it is not yet known how microgravity is perceived by the cell, and it is unknown how cells communicate about the change in the gravity environment, yet it appears that they do. Evidence suggests it is a mechanical mechanism that allows cells to respond to the change of gravity, and the cytoskeleton is at the heart of that adaptation.
It is the intricate cytoskeleton system that plays the primary role of supporting the cellular space. The cytoskeleton is made of three primary types of filaments. Actin filaments are also known as microfilaments. They have a diameter of 5 to 9 nanometers, they are two strands of protein twisted together, and they are pretty flexible structures (Alberts et al. 2008). Microtubules are long and hollow cylinders of tubulin protein; they have an outer diameter of 25 nanometers. Microtubules are long and straight and are anchored on the centrosome (small organelle within the cell). Intermediate filaments are often described as rope-like structures, they have a diameter of about 10 nanometers, and many of them form a mesh-like net throughout the cytosol of the cell. Cytoskeleton fibers are anchored directly and indirectly to the nuclear membrane where the DNA is stored, but also to the cellular membrane.
Bone Cells in Microgravity
We know from Wolff’s Law (Burr and Allen 2014) that the bones are in a perpetual state of remodeling, certain cells are always making more hard bone – the osteoblasts. And at the same time, there are cells that are always pulling calcium out of the hard bone and leaving holes in the bone – the osteoclasts. We need calcium to be that hard bone material that allows us to weight-bear in our upright stance. And we need calcium to be soluble in the bloodstream for many biochemical functions throughout the body. Bones are a balance. When astronauts were found to have microgravity-induced bone loss, molecular biology researchers started looking at osteoblasts and osteoclasts to see what roles each had in this outcome.
The first observation researchers made was that when people go from Earth’s gravity to spaceflight microgravity, the change alters the interstitial fluid flow in the extracellular matrix within the bones, changing the fluid flow around all the bone cells. Also, the connections between bone cells, the cell-cell adhesions, and the extracellular matrix shift physical position. This is due to the bones no longer having the compressive force of weight from being on Earth’s surface, leading to the active remodeling processes changing where the calcium is located.
It appears that in space, there is a decrease in bone formation (osteoblasts are downregulated) more than an increase in bone absorption by osteoclasts. When they looked more deeply at osteoblasts in microgravity, it appeared that the cytoskeleton had undergone a dramatic reorganization on many levels. Microtubules in osteoblasts had shorter and wavier fibers, and there was less tubulin being produced by the cell. Without the fibrous structure, bone cells weren’t able to duplicate at their regular rate. The result is demineralized bone tissue over time.
The Cytoskeleton of Muscle Cells in Microgravity
People face muscle disuse and muscle atrophy when they spend many months in spaceflight. “The antigravity soleus, calf, hamstrings, quadriceps, and back muscles of astronauts during the Skylab mission3 decreased 3.9% to 10.3% after eight days of spaceflight, and with a longer stay in space for six months, up to 20% muscle atrophy was observed in astronauts” (Wu et al. 2021, 2-3).
In normal function, some muscle cells (myoblasts) fuse together to become multi-nucleated muscle fibers. Cultured muscle cells in microgravity failed to fuse, when they would normally fuse in laboratory conditions on Earth. The fusion process of myoblasts relies on the rapid remodeling of the cytoskeleton and, in particular, the construction and reconstruction of actin filaments. Wu et al. (2021) speculate that the altered fusion of muscle cells in space likely involves the disrupted cytoskeleton organization, the connections adhering the cells together are likely disrupted, so the muscle fibers are unable to complete their progression to being multi-nucleated muscle fibers.
Cardiovascular Cells in Microgravity
As already mentioned, body fluids are redistributed in the body when an astronaut goes to space because of the absence of gravity. When they return to the Earth’s surface, astronauts are unable to stand up without fainting because the body cannot get the blood to the high height of the heart and brain. This orthostatic intolerance is thought to be due to many factors, including reduced sympathetic nerve activity. Yet cardiovascular deconditioning, losing strength within the cardiac muscle, and loss of tensile strength in arterial blood vessels, are considered to be the primary cause of this experience. Several studies have reported that the heart muscle itself atrophies during spaceflight. Also, there is a loss of beat synchrony between the cardiomyocytes. There appears to be a disassembly of the microtubules within the cardiac cells, which leads to the heart cells losing their individual contractile strength. And the cell-cell connections between the cardiac cells are disrupted as they fail to beat at the same time, as they normally would.
Wu et al. (2021) also report a decrease in peripheral vascular resistance and decreased vasoconstriction that contributes to postflight orthostatic intolerance. Without the same preflight elasticity in the blood vessels, blood pressure cannot be maintained to support getting blood up to the heart and brain when the astronauts are back on Earth. Endothelial cells within the blood vessels appear to be flatter and have small nuclei in microgravity conditions. These cells have compromised cytoskeleton remodeling with decreased amounts of actin and tubulin. The distribution of where cytoskeleton fibers are found within the cell becomes rearranged in microgravity.
Immune Cells in Space
Astronauts experience a higher risk of infections during spaceflight due to the immunological change caused by the cytoskeleton reorganization in microgravity conditions. There are many factors leading to spaceflight infections, like the enclosed environment, stressors, neuroendocrine disruption, and exposure to radiation from the sun. Yet it is still the impaired activation of lymphocytes that is thought to be the causative factor for deceased immunity (Wu et al. 2021).
One type of lymphocyte, the T cell, is responsible for destroying infectious factors like viruses or even cancerous cells. The microtubules appear shortened and diffused in T cells in space. Along with the cytoskeleton changes, T cells self-destructed at an increased rate, otherwise known as apoptosis. Monocytes, another type of immune system cell, also display severe cytoskeletal changes in space, and this alters their ability to become macrophages that surround and destroy foreign microorganisms. It appears that within their cytosol, the microfilaments lost their organized network and instead were found to be accumulated near the cell membrane. Microtubules were disrupted and disorganized near the nucleus of the cell.
Conclusions of Wu et al. (2021)
The bottom line from Wu et al. (2021) is that the cytoskeleton of cells throughout the body becomes disorganized in the absence of gravity, and cellular tissue slowly atrophies. People have not died in space due to cardiac deconditioning, bone loss, muscle atrophy, and immune system dysfunction, yet perhaps spaceflights have not yet been long enough to lead to these events.
Wu et al. (2021) conclude that individual cells detect and adapt to the mechanical absence of gravity, and that cytoskeleton organization is dependent on gravity to maintain a mechanical equilibrium within the cell. Without gravity steadily relating to the contents of each cell, the loose columnar arrangements of protein synthesis become disrupted. The loose bonds that hold collections of molecules together experience fluid shear forces in the transition to microgravity.
In the bones, osteoblasts no longer receive the input of mechanical tension from the body’s weight, so they no longer build strong bones. Postural muscle cells lose the rigor of their cytoskeleton, which disrupts the cell-cell adhesions needed for strong muscle contractions. And muscle cells seem to be triggered to self-destruct at a high rate in weightlessness, despite people doing resistance-focused exercises. Perhaps most worrying is the loss of structure and function within the cytoplasm of cardiac cells, vascular cells, and cells of the immune system. Cytoskeleton integrity is compromised in prolonged spaceflight and this affects each tissue type uniquely.
Wu et al. (2021) detail the cellular consequences of the absence of gravity, which gives us Rolfers and SI community further insight into the actual effect of gravity on human structure and function. Within human cellular tissue, the cytoskeleton fibers are creating space and are informed by the forces of gravity where to place their strong and flexible structures. Cells detect gravity and even need gravity to produce their proteins and products. Gravity is a mechanical force that affects many body systems: the heart, the blood vessels, the bones, and the immune system found throughout the body. While Wu et al. (2021) kept their descriptions to events within the cell, it is of particular interest that it was the postural muscles that had the greatest muscle mass loss while in space. This offers confirming evidence that what Rolf was saying in the 1970s is correct. In order for us as individuals to have balance in gravity we should direct our attention to postural muscles and the alignment with which we hold our weight-bearing bones.
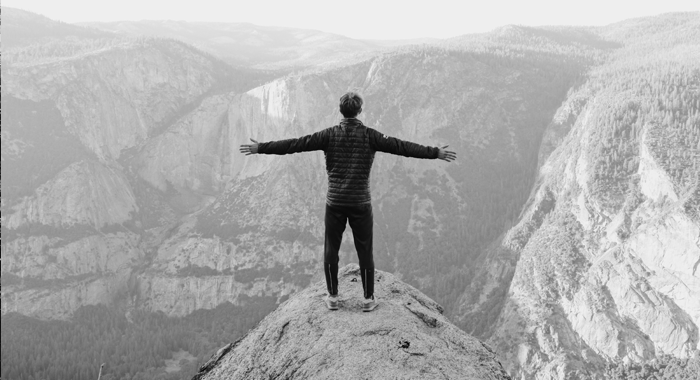
Gravity Expertise
The gravitational field of the earth [sic] is easily the most potent physical influence in any human life. . . .
Gravity is with us from the time of our conception to the moment of death. It is so all-pervading that we cannot sense it, for humans perceive sensory stimulation only as it varies. (We recognize light because there are periods of darkness, sound because we know quiet.) We do not sense gravity, but we do adjust to it. We must.
Ida P. Rolf, PhD, (1989, 30-31).
There is room to grow in our expertise of gravity. Rolf gave us this foundational idea, and she was a woman of her time. The above quote speaks to how all of us are habituated to the downward pull of gravity, unless a person is an SI professional, our attention is rarely brought to think about how the Earth is constantly pulling on us. Now we know that, outside of our conscious awareness, every cell of our body senses gravity.
We have more information at our fingertips than any other generation of people before us, and it is up to us to seek out the research of other disciplines, to be up to date with our understanding of the human form. Rolf deduced her ideas about gravity and human performance at a time when the human body was conceived to be a mechanical machine. The evidence discussed by Harium et al. (2021) and Wu et al. (2021) confirms that there is value in Rolf’s gravity concept. Rolf’s profound and clear idea, that the human body is highly adaptable and operates optimally when organized in the gravitational field, has led to the whole profession of structural integration existing worldwide.
Now, thanks to decades of spaceflight research into the effect of weightlessness on the human body, we have evidence that gravity’s presence does give us instructive direction to our biological system – just as Rolf said. As Rolfers, we tell our clients to feel the downward direction of gravity, then let their heads reach skyward. We invite people to allow their tissues to rest down, enjoy a mindful inhale and feel that natural upward lift where the head and spine are rising. The human body knows how to lengthen upward, and it does this in relationship with gravity.
If the absence of gravity disrupts the cytoskeleton, then the presence of gravity allows for a cytoskeletal balance to organize. If astronauts are plagued with cardiac deconditioning, bone loss, muscle atrophy, constant infections, and disrupted neural functioning, then the presence of gravity on Earth’s surface is stabilizing us more than we readily talk about. Gravity is soothing our hearts by anchoring us to the planet. It holds our blood downward in our bodies, it gives dynamic resistance for muscles to tense against, and it offers vertical direction so that bones can detect where strength is needed in order to be upright. Gravity is a gift that keeps on giving.
Endnotes
1. According to the Guinness World Records (2023), the absolute record for a person being in space was Valeriy Poliyakov (Russia), who spent January 9th, 1994, to March 22, 1995, on the Soviet/Russia Mir space station.
2. Space Oddity performance by Commander Chris Hadfield. https://www.youtube.com/watch?v=KaOC9danxNo.
3. The Skylab mission was the first experimental space station for the United States in 1979. See https://www.nasa.gov/mission_pages/skylab/missions/ for more information.
Lina Amy Hack, BS, BA, SEP, became a Rolfer® in 2004 and is now a Certified Advanced Rolfer (2016) practicing in Canada. She has an honors biochemistry degree from Simon Fraser University (2000) and a high-honors psychology degree from the University of Saskatchewan (2013), as well as a Somatic Experiencing® Practitioner (2015) certification. Hack is the Editor-in-Chief ofStructure, Function, Integration.
References
Alberts, Bruce, Alexander Johnson, Julian Lewis, Martin Raff, Keith Roberts, and Peter Walter. 2008. Molecular biology of the cell, fifth edition. New York: Garland Science, Taylor & Frances Grouop, LLC.
Burr, David B. and Matthew R. Allen (Eds). 2014. Basic and applied bone biology. New York: Elsevier.
Hariom, Senthil Kumar, Akshara Ravi, Gokul Raj Mohan, Harani Devi Pochiraju, Sulagna Chattopadhyay, and Everette Jacob Remington Nelson. 2021. Animal physiology across the gravity continuum. Acta Astronautica 178:522-535.
Rolf, Ida P. 1989. Rolfing: Reestablishing the natural alignment and structural integration of the human body for vitality and well-being. Rochester, Vermont: Healing Arts Press.
Guinness World Records. 2023. Available from https://www.guinnessworldrecords.com/world-records/697059-longest-stay-on-the-international-space-station.
Wu, Win-Tong, Xiao Yang, Ran Tian, Ying-Hui Li, Chun-Yan Wang, Yu-Bo Fan, and Lian-Wen Sun. 2021. Cells respond to space microgravity through cytoskeleton reorganization. FASEB Journal 36:e22114.
Keywords
microgravity; spaceflight; weightlessness; gravity; non-gravity; Ida P. Rolf; astronauts; cardiovascular system; orthostatic intolerance; immune system; bone tissue; muscle fibers; vestibular system; otolith; cellular mechanical equilibrium; cytoskeleton. ■
View all articles: Articles home